Introduction
This chapter provides basic information about bioinstrumentation and electric circuit theory used in other chapters. Many biomedical instruments use a transducer or sensor to convert a signal created by the body into an electric signal. Our goal in this chapter is to develop expertise in electric circuit theory applied to bioinstrumentation. We begin with a description of variables used in circuit theory, charge, current, voltage, power, and energy. Next, Kirchhoff’s current and voltage laws are introduced, followed by resistance, simplifications of resistive circuits, and voltage and current calculations. Circuit analysis techniques are then presented, followed by inductance and capacitance, and solutions of circuits using the differential equation method. Finally, the operational amplifier and time varying signals are introduced.
Before 1900, medicine had little to offer the typical citizen because its resources were mainly the education and little black bag of the physician. The origins of the changes that occurred within medical science are found in several developments that took place in the applied sciences. During the early nineteenth century, diagnosis was based on physical examination, and treatment was designed to heal the structural abnormality. By the late nineteenth century, diagnosis was based on laboratory tests, and treatment was designed to remove the cause of the disorder. The trend toward the use of technology accelerated throughout the twentieth century. During this period, hospitals became institutions of research and technology. Professionals in the areas of chemistry, physics, mechanical engineering, and electrical engineering began to work in conjunction with the medical field, and biomedical engineering became a recognized profession. As a result, medical technology advanced more in the twentieth century than it had in the rest of history combined (Figure 9.1).
During this period, the area of electronics had a significant impact on the development of new medical technology. Men such as Richard Caton and Augustus Desire proved that the human brain and heart depend on bioelectric events. In 1903, William Einthoven expanded on these ideas after he created the first-string galvanometer. Einthoven placed two skin sensors on a man and attached them to the ends of a silvered wire that was suspended through holes drilled in both ends of a large permanent magnet. The suspended silvered wire moved rhythmically as the subject’s heartbeat. By projecting a tiny light beam across the silvered wire, Einthoven was able to record the movement of the wire as waves on a scroll of moving photographic paper. Thus, the invention of the string galvanometer led to the creation of the electrocardiogram (ECG), which is routinely used today to measure and record the electrical activity of abnormal hearts and to compare those signals to normal ones.
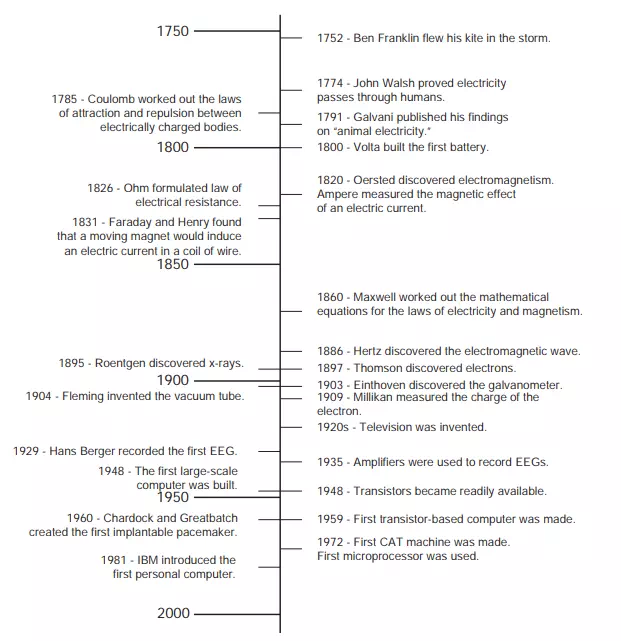
FIGURE 9.1 Timeline for major inventions and discoveries that led to modern medical instrumentation.
In 1929, Hans Berger created the first electroencephalogram (EEG), which is used to measure and record electrical activity of the brain. In 1935, electrical amplifiers were used to prove that the electrical activity of the cortex had a specific rhythm, and in 1960, electrical amplifiers were used in devices such as the first implantable pacemaker that was created by William Chardack and Wilson Greatbatch. These are just a small sample of the many examples in which the field of electronics has been used to significantly advance medical technology.
Many other advancements that were made in medical technology originated from research in basic and applied physics. In 1895, the x-ray machine, one of the most important technological inventions in the medical field, was created when W. K. Roentgen found that x-rays could be used to give pictures of the internal structures of the body. Thus, the x-ray machine was the first imaging device to be created.
Another important addition to medical technology was provided by the invention of the computer, which allowed much faster and more complicated analyses and functions to be performed. One of the first computer-based instruments in the field of medicine, the sequential multiple analyser plus computer, was used to store a vast amount of data pertaining to clinical laboratory information. The invention of the computer made it possible for laboratory tests to be performed and analyzed faster and more accurately.
The first large-scale computer-based medical instrument was created in 1972 when the computerized axial tomography (CAT) machine was invented. The CAT machine created an image that showed all of the internal structures that lie in a single plane of the body. This new type of image made it possible to have more accurate and easier diagnosis of tumors, haemorrhages, and other internal damage from information that was obtained noninvasively.
Telemedicine, which uses computer technology to transmit information from one medical site to another, is being explored to permit access to health care for patients in remote locations. Telemedicine can be used to let a specialist in a major hospital receive information on a patient in a rural area and send back a plan of treatment specific to that patient.
Today, a wide variety of medical devices and instrumentation systems are available. Some are used to monitor patient conditions or acquire information for diagnostic purposes—for example, ECG and EEG machines—while others are used to control physiological functions—for example, pacemakers and ventilators. Some devices, like pacemakers, are implantable, while many others are used noninvasively. This chapter focuses on those features that are common to devices that are used to acquire and process physiological data.
Basic Bioinstrumentation System
The quantity, property, or condition that is measured by an instrumentation system is called the measurand (Figure 9.2). This can be a bioelectric signal, such as those generated by muscles or the brain, or a chemical or mechanical signal that is converted to an electrical signal. As explained in Chapter 10, sensors are used to convert physical measurands into electric outputs. The outputs from these biosensors are analogue signals—that is, continuous signals—that are sent to the analogue processing and digital conversion block. There, the signals are amplified, filtered, conditioned, and converted to digital form. Methods for modifying analogue signals, such as amplifying and filtering an ECG signal, are discussed
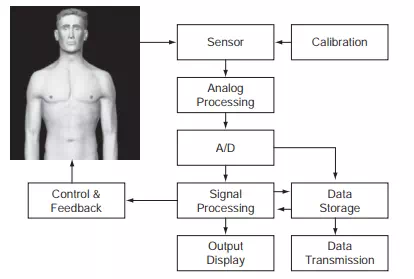
FIGURE 9.2 Basic instrumentation systems using sensors to measure a signal with data acquisition, storage, and display capabilities, along with control and feedback.
later in this chapter. Once the analogue signals have been digitized and converted to a form that can be stored and processed by digital computers, many more methods of signal conditioning can be applied.
Basic instrumentation systems also include output display devices that enable human operators to view the signal in a format that is easy to understand. These displays may be numerical or graphical, discrete or continuous, and permanent or temporary. Most output display devices are intended to be observed visually, but some also provide audible output—for example, a beeping sound with each heartbeat.
In addition to displaying data, many instrumentation systems have the capability of storing data. In some devices, the signal is stored briefly so further processing can take place or, so an operator can examine the data. In other cases, the signals are stored permanently so different signal processing schemes can be applied at a later time. Holter monitors, for example, acquire 24 hours of ECG data that is later processed to determine arrhythmic activity and other important diagnostic characteristics.
With the invention of the telephone and now with the Internet, signals can be acquired with a device in one location, perhaps in a patient’s home, and transmitted to another device for processing and/or storage. This has made it possible, for example, to provide quick diagnostic feedback if a patient has an unusual heart rhythm while at home. It has also allowed medical facilities in rural areas to transmit diagnostic images to tertiary care hospitals so that specialized physicians can help general practitioners arrive at more accurate diagnoses.
Two other components play important roles in instrumentation systems. The first is the calibration signal. A signal with known amplitude and frequency content is applied to the instrumentation system at the sensor’s input. The calibration signal allows the components of the system to be adjusted so that the output and input have a known, measured relationship. Without this information, it is impossible to convert the output of an instrument system into a meaningful representation of the measurand. Another important component, a feedback element, is not a part of all instrumentation systems. These devices include pacemakers and ventilators that stimulate the heart or the lungs. Some feedback devices collect physiological data and stimulate a response—a heartbeat or breath—when needed or are part of biofeedback systems in which the patient is made aware of a physiological measurement, such as blood pressure, and uses conscious control to change the physiological response.