Materials In Medicine: From Prosthetics To Regeneration
Throughout the ages, materials used in medicine (biomaterials) have made an enormous impact on the treatment of injury and disease of the human body. Biomaterials use increased rapidly in the late 1800s, particularly after the advent of aseptic surgical technique by Dr. Joseph Lister in the 1860s. The first metal devices to fix bone fractures were used as early as the late eighteenth to nineteenth centuries, the first total hip replacement prosthesis was implanted in 1938, and in the 1950s and 1960s, polymers were introduced for cornea replacements and as blood vessel replacements. Today, biomaterials are used throughout the body (Figure 5.1). Estimates of the numbers of biomedical devices incorporating biomaterials used in the United States in 2006 include the following:
• Total hip joint replacements: 579,271
• Knee joint replacements: 1,349,641
• Shoulder joint replacements: 49,000
• Dental implants: 1,040,172
• Coronary stents: 1,489,980
• Coronary catheters: 1,648,235
Millions of lives have been saved due to biomaterials, and the quality of life for millions more is improved every year due to biomaterials. The field remains a rich area for research and invention because no one material is suitable for all biomaterial applications, and new applications are continually being developed as medicine advances. In addition, there are still many unanswered questions regarding the biological response to biomaterials and
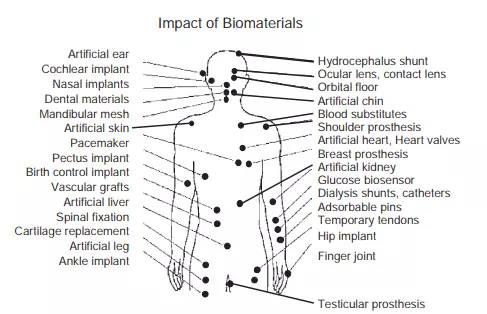
FIGURE 5.1 Biomaterials have made an enormous impact on the treatment of injury and disease and are used throughout the body
the optimal role of biomaterials in tissue regeneration that continue to motivate biomaterials research and new product development.
Over most of history, minimal understanding of the biological mechanisms of tissues meant that the biomedical engineering approach was to completely replace the damaged body part with a prosthetic—a simple, nonbiologically active piece of hardware. As our understanding of developmental biology, disease, and healthy tissue structure and function improved, the concept of attempting to repair damaged tissues emerged. More recently, with the advent of stem cell research, the medical field believes it will be possible to regenerate damaged or diseased tissues by cell-based tissue engineering approaches (see Chapter 6). The notion of a biomaterial has evolved over time in step with changing medical concepts. Williams in 1987 defined a biomaterial as “a nonviable material used in a medical device, intended to interact with biological systems.” This definition still holds true today and encompasses the earliest use of biomaterials for replacing form (e.g., wooden leg, glass eye), as well as the current use of biomaterials in regenerative medical devices such as a biodegradable scaffold used to deliver cells for tissue engineering. While the definition has remained the same, there have been dramatic changes in understanding of the level of interaction of biomaterials with the biological system (in this case, the human body). The expectations for biomaterial function have advanced from remaining relatively inert in the body, to being “bioactive” and not blocking regeneration, to providing biological cues that initiate and guide regeneration. Now there are biomaterials that can initiate a biological response after implantation such as cell adhesion, proliferation, or more excitingly, the differentiation of a stem cell that may one day lead to regeneration of a whole organ.
Due to the complexity of cell and tissue reactions to biomaterials, it has proven advantageous to look to nature for guidance on biomaterials design, selection, synthesis, and fabrication. This approach is known as biomimetics. Within the discipline of biomaterials, biomimetics involves imitating aspects of natural materials or living tissues such as their chemistry, microstructure, or fabrication method. This does not always lead to the desired outcome, since many of the functionalities of natural tissues are as yet unknown. Furthermore, the desirable or optimal properties of a biomaterial vary enormously, depending on where they will be used in the body. Therefore, in addition to presenting general strategies for guiding tissue repair by varying the chemistry, structure, and properties of biomaterials, this chapter includes application-specific biomaterials solutions for several of the major organ systems in the body and for drug delivery applications. This chapter also includes a section on the regulatory approval process and the testing required that play an essential role in establishing and ensuring the safety and efficacy of medical products.
Biomaterials: Types, Properties, And Their Applications
There is a wide choice of possible biomaterials to use for any given biomedical application. The engineer must begin by selecting which general class of material to use. The four basic classes or types of materials are metals, ceramics/glasses, polymers, and composites, which are mixtures of any of the first three types of materials. Natural materials such as animal heart valves are made of proteins that have a repeating polymeric-type structure and thus fall under the polymer category. Every type of biomaterial can be categorized as belonging to one of these four main classes. It is useful to know the types or classes of materials and the basic properties they possess based on their molecular structure when designing a new medical device. The current uses of the various types of biomaterials for medical devices are shown in Table 5.1, and their mechanical properties are shown in Table 5.2.
TABLE 5.1 Materials and Their Medical Uses
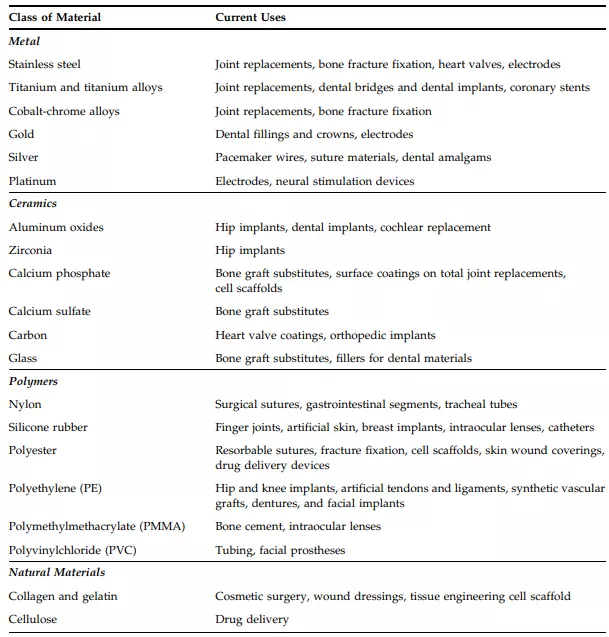
TABLE 5.1 Materials and Their Medical Uses—Cont’d
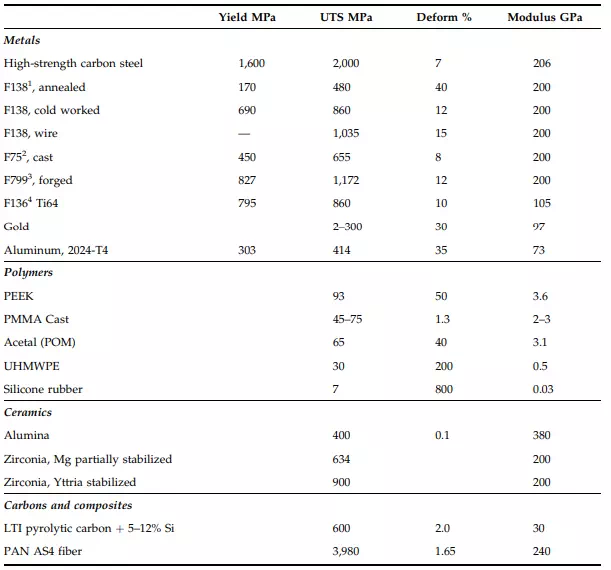
TABLE 5.2 Mechanical Properties of Materials with Literature Values or Minimum Values from Standards— Cont’d
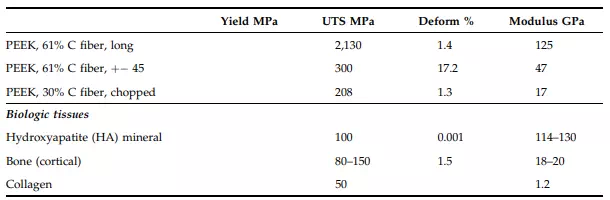
Metals
Metallic biomaterials represent the most highly used class of biomaterials. Metals have high strength and resistance to fracture and are designed to resist corrosion. The main metallic biomaterials in use today can be categorized into three groups: iron-base alloys (stainless steels), cobalt-base alloys, and titanium-base alloys. Many orthopaedic devices are made in part of metal, such as hip and knee joint replacements (Figures 5.2 and 5.3) due to its high strength and ability to resist failure even after many cycles of loading. The implants provide relief from pain and restore function to joints in which the natural cartilage has been worn down or damaged. Plates and screws that hold fractured bone together during healing also are made of metal and are shown in Figure 5.4. Sometimes the metallic plates and screws are retrieved after successful healing, but in other cases they are left in place. Dental root prosthetic implants are also made of metal (Figure 5.5). Other examples of metals used in medical devices and their mechanical properties are shown in Tables 5.1 and 5.2.
Materials selection for a medical device is complicated. The selection depends on a number of factors, including the mechanical loading requirements, chemical and structural properties of the material itself, and the biological requirements. The longstanding use of metals for knee and hip joints, bone plates, and spinal fusion devices is due to the high mechanical strength requirements of these applications and proven biocompatibility in these settings. The advantages of metals over other materials such as ceramics and polymers are that they are strong, tough, and ductile (or deformable, particularly as compared to ceramics). The metal atoms are arranged in a highly ordered crystalline manner yet have nondirectional metallic bonding that allows for the propagation of energy-absorbing dislocations of some of the atoms rather than abrupt, catastrophic cracking. Disadvantages include susceptibility to corrosion, again due to the nature of the metallic bond (free electrons). In fact, the steels that were used in the early 1900s for hip implants corroded rapidly in the body and caused adverse effects on the healing process. This has led to the
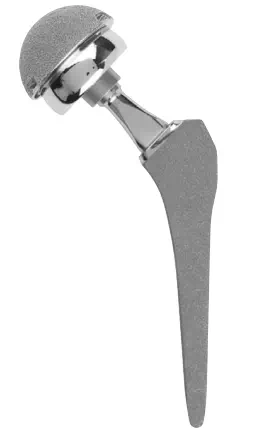
FIGURE 5.2 A typical total hip joint replacement is made primarily of metal. The ball of the femoral hip stem fits into a pelvic acetabular cup that is lined with ultra-high molecular weight polyethylene (UHMWPE) for friction-free motion. Photograph of the PROFEMURW Z minimally invasive hip stem with modular necks courtesy of Wright Medical Technology, Inc.
preferred selection of alloys of titanium or cobalt-chrome for hip, knee, and dental implants. Other typical properties of metallic materials include a high density and much greater stiffness than most natural materials they replace, which lead to undesirable stress shielding. Stress shielding prevents the tissue from being exposed to normal levels of mechanical loading and has been observed after implantation of metal joint replacements to lead to loss of adjacent bone, which requires loading to maintain its mass. Certain metals known as shape memory alloys (e.g., nitinol) can be bent or deformed and still return to their original shape when the stress is released. These metals have found application in eye glasses and coronary artery stents that can be inserted through a catheter while collapsed and then spring into a cylindrical shape once they are pushed beyond the confines of the catheter.
Metallic devices are typically made by investment casting, computer-aided design and machining (CAD/CAM), grinding, or powder metallurgy techniques. The specific steps involved in the fabrication of a medical device will depend on factors such as final geometry of the implant, the forming and machining properties of the metal, and the costs of alternative fabrication methods.
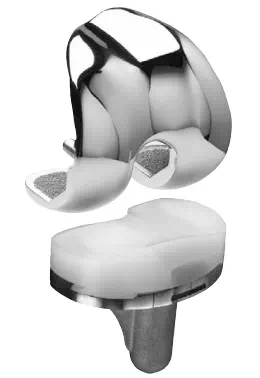
FIGURE 5.3 A metallic artificial knee joint with an ultra-high molecular weight polyethylene bearing surface. Photograph of the ADVANCEW medial-pivot knee system courtesy of Wright Medical Technology, Inc.
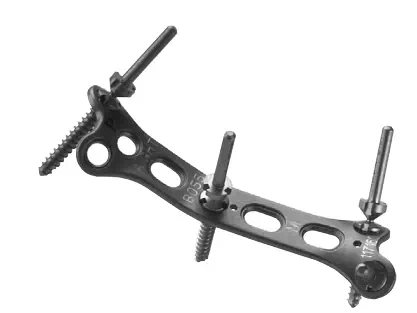
FIGURE 5.4 (a) Metal plates and screws are used to hold fractured bone segments together during healing. Depending on the extent of injury, the plates and screws or rods may be removed when the bone is fully repaired.
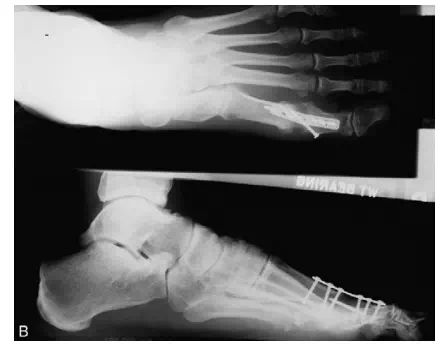
FIGURE 5.4, cont’d (b) Through the use of x-rays, an implanted metal plate with screws can be visualized in this patient’s foot and hand. Photograph of the HALLUW-FIX MTP Fusion System (registered mark of NEWDEAL) is courtesy of Wright Medical Technology, Inc. X-ray courtesy of Wright Medical Technology, Inc.
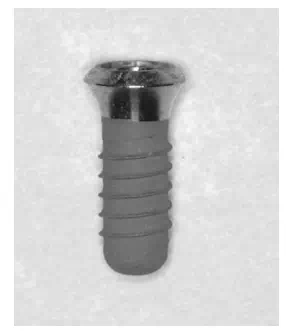
FIGURE 5.5 As an alternative to dentures, patients can have metallic dental root prosthetics implanted to replace each missing tooth. The implant is then topped with a porcelain crown. One advantage of dental implants over dentures is that the implant transmits mechanical forces into the jawbone and stimulates it, resulting in less bone recession over time. Photograph courtesy of Dr. Martin Freilich of the University of Connecticut Health Centre.