The tremendous clinical utility of MRI is due to the great variety of mechanisms that can be exploited to create image contrast. If magnetic resonance images were restricted to water density, MRI would be considerably less useful, since most tissues would appear identical. Fortunately, many different MRI contrast mechanisms can be employed to distinguish different tissues and disease processes.
The primary contrast mechanisms exploit relaxation of the magnetization. The two types of relaxations are termed spin–lattice relaxation, characterized by a relaxation time T1, and spin–spin relaxation, characterized by a relaxation time T2.
Spin–lattice relaxation describes the rate of recovery of the z component of magnetization toward equilibrium after it has been disturbed by RF pulses. The recovery is given by

where M0 is the equilibrium magnetization. Differences in the T1 time constant can be used to produce image contrast by exciting all magnetization and then imaging before full recovery has been achieved. This is illustrated on the left in Figure 12.3. An initial π/2 RF pulse destroys all the longitudinal magnetization. The plots show the recovery of two different T1 components. The short T1 component recovers faster and produces more signal. This gives a T1-weighted image.
Spin–spin relaxation describes the rate at which the NMR signal decays after it has been created. The signal is proportional to the transverse magnetization and is given by
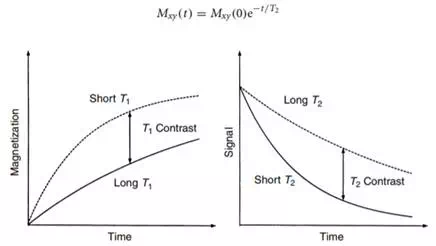
FIGURE 12.3 The two primary MRI contrast mechanisms, T1 and T2. T1, illustrated on the left, describes the rate at which the equilibrium Mzk magnetization is restored after it has been disturbed. T1 contrast is produced by imaging before full recovery has been obtained. T2, illustrated on the right, describes the rate at which the MRI signal decays after it has been created. T2 contrast is produced by delaying data acquisition, so shorter T2 components produce less signal.
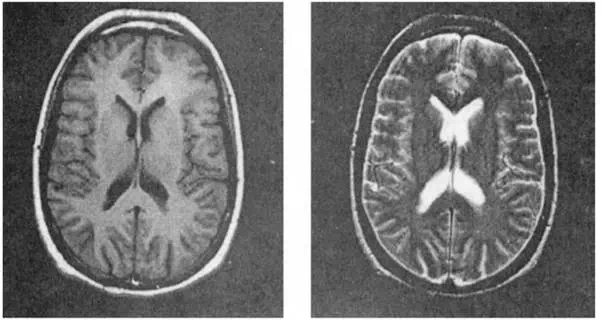
FIGURE 12.4 Example images of a normal volunteer demonstrating T1 contrast on the left and T2 contrast on the right.
Image contrast is produced by delaying the data acquisition. The decay of two different T2 species is plotted on the right in Figure 12.3. The signal from the shorter T2 component decays more rapidly, while that of the longer T2 component persists. At the time of data collection, the longer T2 component produces more signal. This produces a T2-weighted image.
Figure 12.4 shows examples of these two basic types of contrast. These images are of identical axial sections through the brain of a normal volunteer. The image of the left was acquired with an imaging method that produces T1 contrast. The very bright ring of subcutaneous fat is due to its relatively short T1. White matter has a shorter T1 than gray matter, so it shows up brighter in this image. The image on the right was acquired with an imaging method that produces T2 contrast. Here the cerebrospinal fluid in the ventricles is bright due to its long T2. White matter has a shorter T2 than gray matter, so it is darker in this image.
There are many other contrast mechanisms that are important in MRI. Different chemical species have slightly different resonant frequencies, and this can be used to image one particular component. It is possible to design RF and gradient waveforms so that the image shows only moving spins. This is of great utility in MR angiography, allowing the noninvasive depiction of the vascular system. Another contrast mechanism is called T2. This relaxation parameter is useful for functional imaging. It occurs when there is a significant spread of Larmor frequencies within a voxel. The superposition signal is attenuated faster than T2 due to destructive interference between the different frequencies.
In addition to the intrinsic tissue contrast, artificial MRI contrast agents also can be introduced. These are usually administered intravenously or orally. Many different contrast mechanisms can be exploited, but the most popular agents decrease both T1 and T2. One agent approved for clinical use is gadolinium DPTA. Decreasing T1 causes faster signal recovery and a higher signal on a T1-weighted image. The contrast-enhanced regions then show up bright relative to the rest of the image.