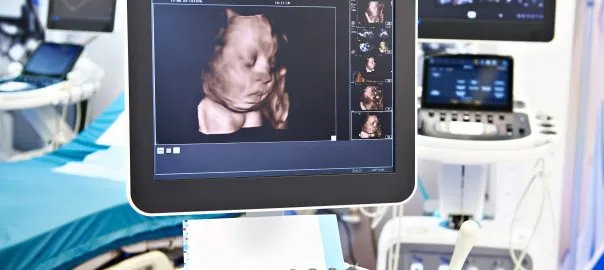
Diagnostic and Therapeutic Ultrasound Imaging
The National Health Service in England carried out 42.1 million imaging tests in the year to March 2017 compared with 40.7 million in the previous year. Plain X-ray radiography was the most common with 22.9 million procedures followed by 9.37 million diagnostic ultrasound scans. Also, computed tomography generated 4.82 million scans compared to 3.36 million magnetic resonance imaging scans.
These imaging modalities demonstrate that ultrasound is more available across a broad spectrum of healthcare services. Ultrasound imaging offers a more cost-effective diagnostic procedure to contribute to the overall diagnosis of the disease state in the patient.
The incorporation of ultrasound imaging in the clinical point-of-care pathway of the patient is to enable the delivery of healthcare products and services in a safe and economical approach compared to traditional X-ray imaging which is not really recommended to be over-used for radiation dosage considerations.
The last 5 years many healthcare organisations have made substantial investments in medical ultrasound imaging. This commitment is driven by the advancement of software technology to be able to produce greater accuracy in analysing ultrasound images thereby delivering the best treatment plan for the patient.
The magic of ultrasound imaging enables healthcare professionals to look inside the human body without being invasive. These high-tech ultrasound machines can diagnose and screen for abnormalities to allow a course of treatment and monitoring.
Since the 1960s ultrasound imaging systems have been widely distributed and used throughout the world amounting to a yearly use of over a billion scans. These portable ultrasound medical devices have been available since the early start of 2000 and after a decade have given way to wireless ultrasound imaging transducers in the form of handheld computers.
During these past two decades, medical ultrasound imaging has contributed to the advancement of theranostics. For example, in the development of ultrasound machines to be used in elastography, contrast agents, resolution imaging and the application of 2-D array transducers. The imaging modality elastography maps the elastic properties and stiffness of soft tissue.
Elastography aims to determine if the tissue mass is hard or soft and will generate information towards a possible cancer diagnosis of certain fibrous tumours. Other applications would include the evaluation of plaque in an artery during stent implantation. However, elastic characteristics cannot be clearly visualised in general ultrasound images.
To circumvent these issues the quantitative measure of elasticity can be assisted by using an acoustic radiation force which is a physical phenomenon resulting from the interaction of an acoustic wave with an obstacle placed along its projection. The force exerted on the obstacle is calculated by the integration of the acoustic radiation pressure over its surface. This radiation force provides controlled deformation of the target tissue.
This action is implemented by an ultrasound imaging transducer via an algorithm to the local tissue position which is accurate to tens of micrometres. These technologies – combined with a high frame ultrasound imaging system – can have a frame rate of 10,000/s which provides high-resolution quantitative elastography.
Other ultrasound technologies use super-resolution imaging by tracking microbubbles in a blood vessel with a high frame system. However, a single microbubble is classed as a scatterer due to the resolution being more elevated than the point spread function of the ultrasound system. The resultant images can provide excellent vasculature networks in the organ. In order to expand ultrasound 3-D imaging, a 2-D array transducer is required which has led to the development of micro-machined sensors.
These 2-D phased array transducers operate in high intensity focussed ultrasound (HIFU) and can facilitate a response to implement abdominal cancer treatment. Also, this approach can be used to treat brain diseases without craniotomy or burr hole trephination.
Furthermore, MRI guidance systems for brain treatment can be used in conjunction with ultrasound technology to apply a non-invasive selective treatment towards brain-related diseases. This technology platform can deliver results in targeted drug delivery aided by ultrasound and microbubbles.
These microbubbles are generated in the time variable acoustic pressure field and will undergo oscillation resulting in the collapse of the bubbles. This process is called acoustic cavitation and occurs close to the biological boundary which is temporarily disturbed.
Research has demonstrated that ultrasound-mediated drug delivery through the blood-brain barrier has been most successful in animal model experiments and consequently extended to targeted brain cancer treatments as well as other parts of the human body.
A new ultrasound imaging technique is called histotripsy. This approach involves the mechanical fractionation of tissue structure by applying a number of short high-intensity ultrasound pulses. The ultrasound intensity used is several magnitudes higher than conventional diagnostic imaging. This pulse sequence is similar to lithotripsy which is used to break down kidney stones.
The action of histotripsy at the fluid-tissue interface will result in localised tissue removal with appropriate sharp boundaries. This approach has been used to remove cardiac tissue in the treatment of congenital heart disease. However, when applied to bulk tissue, histotripsy produces mechanical fragmentation of the tissue site which results in liquefication producing defined boundaries.
Histotripsy has broad clinical applications where precise tissue ablation and removal are needed especially in the treatment of the tumour volume. This technique provides clear-cut lines between treated tissue and surrounding tissue, unlike HIFU which generally uses thermal effect and has a smeared boundary. Accordingly, histotripsy can be an extremely selective tool for cancer treatment.
Since ultrasound imaging does not involve radiation compared to CT scanning: these new developments in ultrasound technology will in the future become more prevalent in the clinical arena especially in emergency medicine. The ultrasound imaging platform will be the chosen diagnostic tool for cardiology, critical care and anaesthesiology.
Ultrasound imaging has become more mobile amongst healthcare professionals by providing a broad range of diagnostic imaging capabilities at the patient location. This proven technology offers a fast, high-quality ultrasound examination at the patients point-of-care. These ultrasound technologies bring many advantages towards patient treatment programmes especially with an increase in patient safety and the improvement of clinician efficiency. This can be clearly demonstrated by using the Edge II which can enhance to accelerate image acquisition to enable the clinicians to make the correct diagnosis of the disease state of the patient.
Future developments in ultrasound medical imaging will be to provide cost-effective solutions that do not compromise image quality. The next generation of ultrasound machines will be automated and mobile to enable effective patient diagnosis and treatment planning.