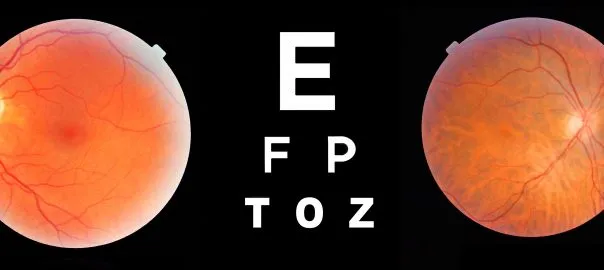
Optic Nerve Imaging in Glaucoma
The ten cranial nerves exit from the human brain including the brainstem and relay information to other parts of the body. One of these cranial nerves is the optic nerve (II) which is 50 mm long and myelinated by oligodendrocytes. It passes through the optic foramen in the sphenoid bone and travels to the eye. The primary function of the optic nerve is to transmit visual impulses from the retina to the visual cortex of the brain. The visual cortex is a part of the cerebral cortex that processes visual information. It is located in the occipital lobe. Hence, the visual nerves run straight from the eye to the primary visual cortex to the visual association cortex. The area where the ganglion cell axons leave the retina does not have any photoreceptors and therefore no visual information is detected in this region. This results in a blind spot in the visual field.
This information channel can be disruptive due to abnormalities of the optic nerve and brain function. The inner nuclear layer of the retina contains the first sensory bipolar cell body which is a ganglion cell layer which transforms the bipolar cell synapses into the optic nerve. Hence, the axons leave the globe at the optic disc to form the optic nerves. Since the 1800s, optic disc photography has been considered the gold standard for optic nerve evaluation.
The basic anatomy of the optic nerve can be considered into four parts:
· The intraocular is about 1 mm in diameter and emerges through the scleral opening;
· Intraorbital is a 25 mm long segment and its function is to communicate between the subarachnoid space around the optic nerve;
· Intracanalicular is 9 mm long and passes through the bony optic canal along with ophthalmic artery;
· Finally, the prechiasmatic is 16 mm in length and is the intracranial segment in the suprasellar cistern region.
The imaging of the optic nerve is vital to the diagnosis of glaucoma where the optic nerve is damaged by pressure due to the fluid inside the eye. In the United States, more than three million Americans are living with glaucoma, and 2.7 million are aged 40 and over. By 2020 it is predicted that 80 million people in the world will develop glaucoma.
The optic nerve is composed of approximately 1.5 million axons that connect the retina to the visual parts in the brain. Most of the axons are about 1 µm in diameter; about 10% of them are 2-10 µm in diameter altogether a nerve fibre is about 3-4 µm thick. In comparison to 120 million photoreceptors in the retina, the signal becomes immensely intensified. Consequently, these axons are like a bundle of fibre optic cables and are extensions of the retinal ganglion cells.
During the stages of glaucoma, the retinal ganglion cells and axons suffer damage which creates a loss of function of the optic nerve that can result in visual field deficits.
The technology platform of optic nerve imaging has undergone several advancements over the last decade especially in the area of glaucoma diagnosis. For example, the application of optic nerve photographs including stereo disc-photography. This approach provides imaging data about the state of the optic nerves and can be compared to other high-resolution photographs. The technique stereo disc-photography allows the health professional to detect any changes from the last photographs.
Currently, more advanced optic nerve imaging techniques have allowed the quantification of specific parameters related to the optic nerves and retina. The disadvantage of optic nerve photographs is that it does not provide quantitative information. The advantage of the advanced techniques allows for quantitative data. The goal of these techniques is to measure the thickness of the nerve fibre layer which is part of the retina that contains the axons of the retinal ganglion cells. This approach is used to determine if the patient has glaucoma due to the reduction of the retinal nerve fibre layer.
These advanced imaging techniques include:
Optical Coherence Tomography (OCT): This technique measures the reflection of laser light to generate a 3-D reconstruction of the optic nerve. The most recent advances of OCT include optical coherence tomography angiography (OCT-Angiography), which is used to measure the blood flow to vessels surrounding the optic nerve and the macula. This research indicates that the optic nerves are vulnerable to changes in optic nerve blood flow and this measurement can be used to help in the diagnosis of the disease state of the eye.
The other imaging technique Heidelberg Retina Tomography (HRT) is used to produce a 3-D representation of the optic nerve. This diagnostic procedure is used for precise observation and documentation of the optic nerve head which is paramount in the diagnosis and management of glaucoma. The laser is focused on the surface of the optic nerve to generate the image. During this process, the eye is not damaged by the laser and HRT is able to produce images in the deeper layers of the optic nerve. The type of damage that glaucoma causes in the optic nerve is called cupping. When the cells die in the optic nerve, they leave a small cup in the nerve. Therefore, the imaging information will be able to calculate the dimensions of the cup. Also, HRT can be used to calculate the change in area of the optic disc including the volume of the cup over a period of time.
Nerve Fibre Analyser (GDx) uses laser light to measure the thickness of the nerve fibre layer to detect glaucoma. The earlier glaucoma tests centred on measuring eye pressure or measuring the effect that glaucoma has on your overall visual field. During the test, the thickness of the nerve fibre layer is compared with the nerve fibre layer of normal eyes. The GDx maps the nerve fibres and compares them to a database of healthy glaucoma-free patients. A thinning of the fibres indicates glaucoma. The GDx provides good results about the status of the optic nerve: having more advanced instruments are available such as Optical Coherence Tomography (OCT).
Another technology uses the principle of confocal Scanning Laser Ophthalmoscopy (SLO), which is based on the near-infrared diode laser (675 nm) beam to scan the posterior pole. This imaging platform works by detecting the reflected light by using the confocal photodiode which is conjugated to the retinal plane. During this process, the confocal filter only records the light reflected from the narrow spot which is illuminated by the laser to generate stereoscopic high-contrast digital images of the optic nerve. These images can be produced with fluorescein: by changing the laser wavelength, this will enable a selective examination of different tissue depths.
The advantages of using SLO is that it generates high magnification of fine structures within the eye. It also gives a high frame rate to allow a more accurate diagnosis of the retinal structures.
Furthermore, a 3-D tomograph can be produced of the retina to provide information on the thickness. These optical slices are created in the digital mode by obtaining 32 consecutive and equidistant optical section images. The primary use of SLO in the clinical setting has been for the assessment of the surface contour of the optic nerve head, especially in glaucoma.
The diagnostic imaging modalities such as computed tomography (CT), magnetic resonance imaging (MRI), X-ray and ultrasound look at the structure or anatomy in the region of interest. However, Opto Acoustic Imaging evaluates the structure by fusing the information about functionality around its location. This approach works by the combination of anatomic and functional diagnostic imaging which is facilitated by the use of laser optics and conventional ultrasound in real time in order to generate high-resolution contrast images.
Opto Acoustic Imaging can be used to evaluate the presence of tumour neoangiogenesis by detecting new blood vessels produced by malignant tumours. These blood vessels are used by a tumour to supply nutrients and remove waste products. However, these malignant masses cannot grow larger than 2-3 mm without generating neovessels.
The parameters of the laser wavelength are set to enable visualisation of relative amounts of oxygenated and deoxygenated haemoglobin within the vasculature and surrounding tissues.
Inside the tumour there are vessels which supply and drain from a malignant tumour: the haemoglobin gives up its oxygen to the rapidly growing cells and becomes deoxygenated. The combination of haemoglobin concentration and its relative oxygenation has been shown to provide good diagnostic accuracy. Malignant tumours have increased blood (haemoglobin) concentration with relatively decreased oxygen content. Benign growths have variable blood (haemoglobin) concentration with relatively more oxygenation.
Functional information has direct significance to tumour pathophysiology and contributes to the treatment plan of the patient. This is dependent on the nature of the malignancy and whether biopsies are needed to be obtained for further study.
Opto acoustic imaging may permit the identification of tumours as small as 3 mm and this demonstrates the ability to see submillimetre vascular structures. Early detection is essential because biologically advanced tumours are more capable of metastasis.